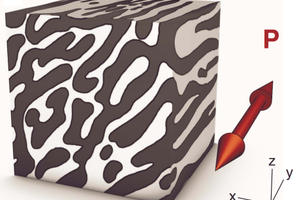
If Moore’s law continues, electronic devices will consume more than half the world’s energy budget within 20 years. To prevent that, we need a fundamentally new material.
One of the extraordinary features of the microelectronics revolution is its ability to scale, a featured captured by Moore’s Law. That has led to a rapid and massive increase in computing capacity—today’s top-of-the-range smartphones have the computing power equivalent to the world’s most powerful supercomputers from the early 1990s. Tomorrow’s smartphones will be even more powerful. But there is a problem in the offing. As powerful computers become more widespread, the amount of power they consume will increase. If Moore’s exponential law continues, electronic devices will consume more than half the planet’s energy budget within a couple of decades. That’s clearly unsustainable. So what to do?
Today we get an answer of sorts from Nicola Spaldin, a materials scientist at ETH Zurich, in Switzerland. Spaldin argues that materials scientists can save the planet, and their solution will be in the form of a fundamental breakthrough that changes the way we think about information technology and the way we use it. She makes the argument—and points to one or two potential avenues for this breakthrough—in a highly entertaining paper.
Spaldin begins by showing how the human civilization has been shaped by breakthroughs in materials science. The discovery of composite materials such as fiber and resin allowed humans to attach blades to sticks to create knives and axes. The remarkable discovery of smelting techniques, probably in stone age pottery kilns, led to the bronze and iron ages. That caused radical changes in agriculture and led to the establishment of cities and even countries. Metal technology also led to Important changes in weapons technology and ultimately, around 4,000 years later, to the industrial revolution.
Later, the discovery of the electron led to the development of the vacuum tube, the solid state transistor, and to microelectronics in general. The ultra-pure silicon required for modern electronics was initially developed for high-frequency radar receivers in the Second World War. Each of these breakthroughs in materials science changed the world and the way we interact with it. But none of them were planned and much about the way of life that preceded them was lost when these changes occurred.
Spaldin argues that something similar will be required to overcome the silicon energy crunch. We cannot continue with silicon, so what will replace it? One possibility may come from Spaldin’s own research on multiferroics—materials that have both ferroelectric and ferromagnetic properties. Usually, the only way to change a material’s magnetic properties is with a magnetic field. But Spaldin and others have shown how to change the magnetic properties of multiferroics with electric fields.
That has significant implications. A great deal of silicon-based information processing and storage relies on magnetic properties that must be manipulated with magnetic fields. The ability to do that more efficiently with electric fields is potentially transformative. “Replacing the magnetic fields in our existing magnetism-based technologies with electric fields offers tremendous opportunity for energy savings, miniaturization, and efficiency,” she says.
Multiferroics have other useful properties. Inside these materials, ferroelectric dipoles can line up with different orientations. Dipoles that are aligned form regions called domains and the borders between these domains turn out to be interesting. Spaldin says these borders form conducting channels than can be moved and rearranged using electric fields. “This has potential application in novel memory or information processing architectures,” she says. The surface of these multiferroic materials also have curious electronic properties that can be manipulated to catalyze reactions such as water splitting. “Our new multiferroic materials are poised to enable new device paradigms, and in turn entirely new ways of designing technologies,” she says. “Perhaps we are about to enter a new Multiferroics Age?”
Gone would be our reliance on silicon and instead we will be dependent on an industry producing erbium manganate or yttrium manganate or bismuth ferrite and a new generation of highly energy efficient information-processing devices. Spaldin is not holding her breath. There are many factors that determine the future of technology and no way of predicting how they will pan out. Multiferroics is one possibility but there are surely many others. And this is her main point. That history clearly shows that the long-term future is never a straightforward extrapolation of today. Instead, disruptive ideas change the world. And the key is to create an environment in which this disruption can happen. The idea that materials scientists will create this revolution is somewhat tongue-in-cheek, however. It will, of course, be physicists who do the important work (cough). She ends her argument with a passionate plea to governments, funding agencies and university administrators.
“The true breakthroughs that will change the course of history will not come from initiatives to improve existing materials or devices, or to advance technologies that have already been identified,” she says. “Instead, they will come from off-beat individuals or small teams of fundamental researchers pushing the boundaries of knowledge in directions for which there is not yet an application.” In other words, fundamental research will pay off in silver dollars—if it is carefully nurtured.
Quelle: MIT Technology Review
Kommentare
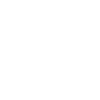